The introduction of the K computer is about to open a path for reproducing the behavior of a biomolecule under conditions close to those of an actual intracellular environment, which has been considered difficult due to the limitations of hitherto computational capabilities. The aim of Theme 1, "Simulations of biomolecules under cellular environments" (leader: Yuji Sugita, Principal Investigator of RIKEN), is to go from molecular level computations to "whole-cell simulation", which is directed to understanding and predicting biological phenomena. Today, we are hearing from six investigators who are undertaking research in the simulation of biomolecules under cellular environments, which is key to achieving this goal.
On the basis of a loose collaboration, the theme of each investigator is tackled
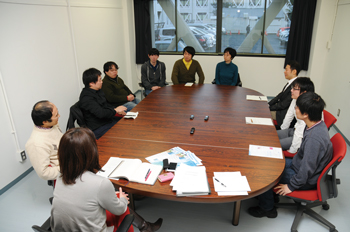
●What type of research are you currently undertaking in Theme 1?
Yu: The aim of the Sugita team is to reproduce, in a computer, the behavior of biomolecules under cellular environments, through large-scale molecular dynamics calculations using the K computer. In this process, we have a bacterium called mycoplasma as the target, and we have created a cell environment model at an atomic level, in which almost all the proteins and metabolites involved in its metabolic network coexist at concentrations that are close to those inside an actual cell. Currently, we are carrying out all-atom molecular dynamics simulations using this model. The interior of a cell is extremely crowded with biomolecules, and there are a large number of proteins, small molecules, ions, and so on, in a melee, similarly to a packed commuter train. What type of dynamics and interactions the proteins and small molecules display in such a packed environment is an important question in biology as well, but it is not well understood, due to the difficulties in obtaining experimental measurements. The objective is to elucidate this aspect.
Iwamoto: What our team is doing is modeling and simulation of intracellular signal transduction pathways, which take the intracellular environment into consideration. A cell responds suitably to a change in the environment. This response is called signal transduction, which pathways are biochemical reaction networks among a number of intracellular proteins. A large number of such signal transduction pathways exist inside a cell. Well known among them is the epidermal growth factor (EGF) signal transduction pathway. It has the role of receiving EGF ligand stimulation from the external environment communicating the information to the cell interior, and prompting the cell to grow, divide or differentiate. We are currently undertaking cell simulations pertaining to the networks in this pathway. The cell simulation is a method for simulating a cell virtually in a computer, and one important feature of our method is that the high computational capabilities of the K computer are utilized so that the cell shape and structures are included and diffusion, collision, reaction fluctuation, and so on, are represented at the molecular resolution.
Kamiya: Intracellular signal transduction is mainly carried out through phosphorylation reactions by several kinases. The reaction activity of a kinase is a chemical process that is key to intracellular signal transduction, and elucidating the molecular mechanism of reaction activity in a cellular environment is important in establishing a molecular platform oriented toward understanding and control of intracellular signal transduction. For this purpose, our team is carrying out research and development related to reactivity analysis of kinases in signal transduction pathways. As mentioned by Dr. Yu, the interior of a cell is an extremely crowded environment. In order to elucidate what kind of chemical reaction is occurring in such a crowded environment and how different it is from a conventional reaction occurring in water, we are performing analyses using the QM/MM method, which combines quantum chemical (QM) computation and molecular mechanical (MM) computation.
Kanada: We are using the coarse-grained molecular dynamics simulation program (CafeMol) which has been developed by our research team to carry out research focused on the MAPK system phosphorylation cascade (a series of reactions), which is a representative model for signal transduction pathways. As already mentioned by Dr. Iwamoto and Dr. Kamiya regarding signal transduction, in the mammalian MAPK system cascade, upon stimulation from the exterior, the upstream protein MEK1 (MAPKK) activates the downstream protein ERK2 (MAPK) by phosphorylating it, leading to a transmission of the signal, finally the signal is transmitted to the interior of the nucleus. However, the details of the molecular mechanism for phosphorylation are not understood, and need to be elucidated. Therefore, based on the individual monomeric structures of MEK and ERK, which have been resolved experimentally, we are investigating the MEK-ERK complex structure and the dynamics of its formation. Another target is related to the ERK protein translocated into the nucleus after phosphorylation. Applying coarse-grained simulation, we would like to elucidate the dynamical behavior of the ERK protein in an intranuclear crowded environment where chromatin is present in high concentration.
Ishida: We are dealing with nucleosomes. The DNA from eukaryotes, including humans, has a fundamental structure called a nucleosome, which is DNA wound around a core of proteins called a histone, and many of them are aligned to form a chromosome, which is housed inside the nucleus in a compact form. This nucleosome is deeply involved in gene regulation, and known to change structure or position in relation to DNA metabolism such as transcription, replication, and repair. In order to elucidate the mechanism of movement, structural stability and so on, of the nucleosome, we are using total atomic models to calculate the extent of DNA winding and unwinding around the histones in each of the systems including conventional canonical histones and variants that constitute the nucleosome. More specifically, we simulate a slow pulling and peeling of the DNA and use the K computer to calculate the structure distribution in so doing, called a free energy profile in expert terms, i.e., whether the free energy of the pulled and peeled DNA is high or low. We are trying to go as far as seeing, at the total atomic level, how different the ease of peeling becomes when a component of the nucleosome is changed.
Kokabu: As explained by Dr. Ishida, the intranuclear DNA has a nucleosome structure in which DNA is wound around histone proteins, and adopts a structure in which several nucleosomes have condensed. In our laboratory, we are undertaking research regarding the functional dynamics of these intranuclear DNA-binding proteins, using molecular simulation, while linking with experimental systems. In this process, we have been undertaking program development for the coarse-grained MD-SAXS method. The SAXS (small-angle X-ray scattering method) is a method in which a protein in solution is irradiated by X-rays, and the overall shape of the molecule is measured from its scattering pattern, and it allows a protein structure that fluctuates to be seen. Meanwhile, MD (molecular dynamics) simulation can predict, on the total atomic level, the behavior of a biological macromolecule such as a protein by solving an equation of motion. However, in the case of a gigantic molecule such as the nucleosome, a total atomic MD simulation would require too much computation time, so that a coarse-grained (CG) MD is used, in which an amino acid is substituted with a particle, to predict the structure, and from this result, the SAXS scattering pattern is computed theoretically. The computation result and the SAXS experimental data are compared and examined; if they correspond, then the simulation result can be deemed appropriate.

※Places that are in bold below is part of the long version published only on the Web version.
●You are doing research and development on your respective themes. Are there any collaborations among you?
Yu: We are collaborating in the sense that we are looking at the same target with different computational precisions. For instance, Dr. Kanada is engaged in the prediction of complexes between MEK and ERK, and we are dealing with the same system as well. Since the time-scale we can deal with differs depending on the level at which coarse-graining is used, a multi-scale collaboration and cooperation is possible, such as, for example, we predict a rough complex structure with the coarse-grained simulation from Dr. Kanada and his team, and we refine this structure with a higher precision simulation called all-atom molecular dynamics.
Kamiya: There are things that cannot be seen until you observe over a long time, and things that cannot be seen unless you observe in detail, so, various methods must be selected and combined according to the target or the situation. In addition, there are systems for which the whole can be understood only when observed with various methods. For instance, understanding in regards to membranes also progresses by observation with a coarse-grained model first, with a classical MD for detail, and with the QM/MM method for finer detail. This truly is multi-scale. However, the barrier between classical MD and QM/MM is not that high; rather, all-atom MD and coarse-grained MD seem farther apart, giving the feeling of multi-scale.
Kanada: Since computational costs decrease dramatically compared to all-atom MD, coarse-grained simulation, which handles an amino acid as a particle, is certainly nice; however, the accuracy of the force fields (particle-particle interactions) might be problematic. In order to solve such a problem and fill the gap between total-atom and coarse-graining methods, our laboratory has been developing the AICG model (atom interaction-based coarse-grained model), which considers the dependency on the amino acid sequence and the secondary structure of a protein. We used this model to perform a MEK-ERK complex structure prediction, resulting in the obtained structures being very similar to those from the total atomic approach by Dr. Yu and his team. Going forward, we need to demonstrate the appropriateness (validity) of our predicted structures by collaborating with an experimental group. In addition, our research is also related to Dr. Kamiya's group as well. A simulation with CafeMol requires a wild-type (reference) structure obtained by experiments; however, the post-phosphorylation wild-type structure of MEK alone has not been obtained experimentally. Thus, we are performing MEK-ERK complex formation simulations based on the structure of phosphorylated MEK that Dr. Kamiya's group derived by all-atom MD simulation.
●Tell us about this fiscal year's progress situation, difficulties, etc.
Yu: Large-scale simulations are almost complete, and this fiscal year, I am mainly doing analysis of the obtained data. While molecular dynamics simulations can provide time-series data about where an atom was and when, absolutely nothing can be learned by just staring at these coordinates. You need to use the data to analyze how a protein is diffusing in the cytoplasm, where a metabolite is distributed in large quantities and how it is moving, etc. That being said, since the amount of data is an enormous amount of tens of terabytes, in order for the needed information to be extracted from there and visualized, I had to write an analysis program of my own, which I am struggling with.
Kamiya: The situation for me is that I am just beginning, after joining in 2013. An important theme for the current fiscal year was program development to run the QM/MM program on the K computer, which I have now completed. I have started undertaking computation related to GTP hydrolysis reactions in Ras-Gap protein complex as a chemical reaction in a molecular crowding environment.
Kokabu: The development of the coarse-grained MD-SAXS method is almost complete, and I am currently performing coarse-grained MD of nucleosomes under various simulation conditions. I struggled with the development of the coarse-grained MD-SAXS method. For instance, in the calculations of SAXS profiles, hydration layers present around a protein must be handled together. It was very difficult to validate the appropriateness of the handling of the hydration layers while making comparisons with hydration layers in all-atom MD.
Iwamoto: This year, I struggled with collecting experimental data. A cell has a three-dimensional structure in reality, but a microscope image can be seen only in two dimensions. Therefore, it was necessary to reconstruct three-dimensional information from slice images, as is done in MRI. However, researchers who do such experiments could not be found nearby easily.
Kanada: As mentioned earlier, a structure prediction of the MEK-ERK complex by CafeMol was performed, and a structure very similar to that from the all-atom approach was obtained. In the future, the complex structure obtained by our simulation needs to be validated by collaborating with an experimental group. In addition, we are proceeding with investigating the dynamics of the ERK protein in an intranuclear crowded environment, through coarse-grained simulation using the K computer.
Ishida: What I struggled with this fiscal year was to port a program to the special architecture of the K computer. In the beginning, I commuted several times to Kobe. Now, having cleared that, I have reached a point where I am just running it.
Undertaking research with a dream
●What is your impression after using the K computer?
Ishida: We are doing a large computation using 4,800 nodes for an all-atom simulation of peeling a nucleosome consisting of approximately 500,000 atoms. The calculations simulate the behavior of about 50,000,000 atoms in total. Such a simulation is indeed impossible if not for the K computer, and I really have the feeling of doing cutting-edge computation, which could not be carried out so far. While we are currently in the middle of analyses, we are starting to learn about what is needed in order to do high-precision analyses through ultra-large system simulations.
Yu: Even in such an extremely small bacterium as mycoplasma, when you try to include all the proteins related to a metabolic network at realistic number density ratios, the total number will end up being about 1,500 at least. Since the simulation of one to a few large proteins is common at a conventional laboratory level, I indeed think this research would be impossible without the K computer. In terms of impression after actual use, in addition to the fact that it is crowded with jobs, determining an optimal number of nodes required a bit of trial-and-error. If the number of nodes secured is too large, the wait time becomes extremely long; conversely, if the number of nodes is too small, although jobs can be placed at a good pace, the trade-off is that the time available for simulation is shorter. I struggled to find the most balanced number of nodes in the beginning.
Kamiya: The K computer is indeed attractive for handling large systems. Regarding MD, we are also using the molecular dynamics simulator "GENESIS", which is used by Dr. Yu's group as well. It is extremely efficient, and helpful to us. The ability to run MD of a large-scale system at high-speed is indeed important. As for QM/MM, while it can be run, there is the fact that the input has become very complicated, and there are many failures, which is an issue. In addition, since job submission has to be reiterated several times, the wait time to do so is long, which is a real problem.
Kanada: When investigating the dynamics of the ERK protein in an intranuclear crowded environment, we are including in the system a chromatin structure consisting of 20 nucleosomes to perform the calculations. My impression is that, since this is an extremely large system for coarse-grained simulation by CafeMol, utilizing the K computer is extremely helpful to proceed with large-scale calculations efficiently and systematically.
Iwamoto: I too think that the ability to look at large systems in fine detail is what makes the K computer attractive. I am dealing with a whole cell. Ideally, I would like to bring the grid size to the size of one protein molecule, i.e. about 5 nanometers, but there is not enough memory in PC clusters such as those in conventional laboratories. On this point, the K computer allows you to proceed with no problem at all.
Kokabu: In my case, since I use coarse-grained simulation, the to-be-computed system itself is not that large, but in order to examine various conditions, I am doing simulation with 90 conditions for one nucleosome. In addition, even for coarse-grained simulation, enough conformational space cannot be searched in only one round, and the same computation is repeated about 100 times. I feel that using the K computer is extremely important for this purpose as well.
●Going forward, what type of research would you like to carry on?
Ishida: I have been doing simulation research on biological macromolecular systems that exceed 1,000,000 atoms. Calculators have been developed, which possess high computational capabilities, such as those of the K computer, and simulation accuracy also has been increasing dramatically compared to the past. In this context, I would like to keep my interest in simulation techniques, and carry out research that nicely links the best part of coarse-grained simulation with the best part of all-atom simulation. In addition, I think that ultimately connecting with drug development and such, may be a theme in simulation research, but I personally would like to carry on steadily with fundamental research.
Yu: In contrast, I would like to expand in the direction of medicine and drug development. The research I am currently undertaking has strong basic science aspects, and while this obviously remains important to me, in the future, I would like to contribute to the development of drug design processes that take the cellular environment into account. Currently, computer-assisted drug development focuses mainly on the affinity between a protein and a drug; to this, I would like to add the influence of the intracellular environment. For example, intracellular water has different viscosity and dielectric constant from pure water, and I believe there is also a significant influence on the binding affinity between a target and a drug. If a general cellular environment model could be built by gathering such findings, wouldn't it lead to a more realistic drug development process?
Kamiya: My "root" being chemistry, I would like to continue by properly handling the chemical reactions upon which the field of life science is based. While setting my aim in such a direction, I would like to contribute to society though drug development, or the like.
Kanada: I would like to reproduce the behavior of large systems by utilizing the simulation program of "CafeMol". I am thinking that whole-cell simulation will also become possible with "CafeMol" in the future. By running high performance calculators such as the K computer over an extended time period, using not only protein models but also DNA and lipid models, it should be possible to simulate an entire cell. While my current main targets are proteins related to signal transduction, I would like to contribute also to research on systems including DNA and lipids.
Iwamoto: To be brief, I too would like to achieve whole-cell simulation. However, I do not mean coarse-grained simulation; I would like to achieve whole-cell simulation including all of the numerous functions that a cell possesses in order to respond to various stimuli from the environment, such as those involved in signal transduction and metabolism. About two years ago, an article came out about an American research group having simulated all the mycoplasma reaction systems. Progress is also being made in whole-simulation research with Escherichia coli and the like. I would also like to proceed in such a direction.
Kokabu: We happen to collaborate with molecular biologists in the experimental field, and when we learn that a prediction by our simulation turned out to be correct through an actual experiment, we are sometimes overjoyed. For such reasons, I would like to continue placing an importance on collaboration with experimenters. I think that findings through simulation are also extremely useful to molecular biologists conducting experiments. I would also like to do research that can support experimental projects, through simulation.